Assimilate Allocation and NMR
Our research aims a better understanding of the relationships between assimilate supply, metabolism and seed architecture, thereby uncovering mechanisms for improved traits in major crop plants.
We investigate growth processes in seeds and specific physiological features (e.g. assimilate translocation, distribution of metabolites and storage products, pattern formation). The plant seed is regarded as living multi-organ system. Its functionality relies on interactive processes among tissues/sub-organs. While current omics-approaches try to dissect the living plant into its (thousands of) components, our attempt is to characterise their in vivo interplay.
We develop topographical, in vivo approaches to identify key factors determining the flow and partitioning of assimilates into starch, lipids and storage proteins in the developing seed. Preferred plant models are cereals (wheat, barley) and oil crops (oilseed rape).
Our approaches crucially rely on novel, non-destructive and imaging procedures, based on nuclear magnetic resonance (NMR), infrared spectroscopy and optical sensors. Additionally, we make use of classical biochemical tools like mass spectrometry and molecular biological tools.
scroll top
Projects
We apply nuclear magnetic resonance imaging and high-resolution analytic technologies to study seed performance in vivo. The allocation of assimilates and metabolic regulation of growth and storage processes is our current focus. Preferred plant models are crops like barley, wheat, maize and oilseed rapeseed. In order to improve versatility of our integrative approach, we also working on Arabidopsis and other plants in the frame of collaboration projects with other universities.
Assimilate supply and allocation routes
Mechanisms underlying the delivery of assimilates to the developing seed are of highest relevance in crop research. The transport route of assimilates is hidden from human eyes and is challenging for investigation. We established non-invasive imaging technology based on nuclear magnetic resonance (NMR), allowing to visualize sucrose allocation along the path from source to sink and especially within the living seed itself.
Non-invasive imaging/monitoring provides information on tissues involved in transport processes, dynamic and spatiotemporal pattern of assimilate import, helping to link developmental and molecular events during seed growth. We apply an integrative approach which includes mass spectrometric biochemical analyses, metabolic modelling, chemical imaging based on infrared spectroscopy and optical microscopy. Such combination represents a powerful platform for the comprehensive study of seed filling in crops (Melkus et al., Plant Biotechnology Journal 2011; Rolletschek et al., Plant Cell 2011; Borisjuk et al., Plant Journal 2012; Rolletschek et al., Plant Physiology 2015, Munz et al., New Phytologist 2017; Radchuk et al., JExpBot 2017).
In current DFG projects, we investigate the functional role of sugar transporters (SUT-, SWEET-families) for seed development and filling.
In laufenden DFG-finanzierten Projekten untersuchen wir zum Beispiel die Rolle von spezifischen Zuckertransportern (SUT-, SWEET-Genfamilien) für die Samenentwicklung in Getreiden.
Adjustment of central metabolism to the hypoxic environment inside the seed
The hypoxic state comes about whenever the capacity for oxygen diffusion is restricted, so that the concentration of oxygen available falls below the level required for cellular metabolism. These conditions apply frequently to the developing seed (for review see Rolletschek H. (2012). “Hypoxia – a phenomenon which shapes seed metabolism”. Habilitation at the Naturwissenschaftliche Fakultät, Leibniz Universität Hannover/Germany). Seed hypoxia is not only because of high respiratory activities but mainly due to the low void space/porosity of seed tissues, and thus limited diffusive oxygen uptake (Verboeven et al., New Phytologist 2013).
In current work, funded by DFG, we are investigating the implications of internal hypoxia for assimilate uptake and partitioning in the developing maize kernel. In particular we work on the functional role of specific metabolic enzymes (PPDK; Lappe et al., PNAS 2018), the metabolic heterogeneity of endosperm (Rolletschek et al., 2017 In: Maize Kernel Development), phytoglobins and signalling components using a number of transgenic and mutant plants.
Metabolic architecture of developing seeds
Our idea is that in vivo metabolic fluxes are locally regulated and connected to seed architecture. In cereal grains, the isolation of the filial from the maternal tissues creates a complex metabolic system, involving a tripartite interaction between the pericarp, endosperm, and embryo. These three grain components in effect form an interactive system of autonomous organs, each following their own genetic programs. To specify whole-grain metabolism at the level of its component tissue parts, we are applying spatially resolved molecular, biochemical and physiological analysis of metabolic activities as well as metabolic modelling approaches.
Recent findings quantified the in vivo metabolic contributions of distinct seed organs and suggest the presence of a mechanism(s) able to ensure metabolic homeostasis in the face of short-term environmental fluctuation (Rolletschek et al., Plant Physiology 2015).
In oilseed rapeseed (Brassica napus), noninvasive NMR-based imaging has previously demonstrated the establishment of steep gradients in lipid accumulation (Borisjuk et al., Progress Lipid Research 2013). We have been investigating how metabolism in the distinct seed organs of B. napus (seed coat, endosperm, inner and outer cotyledons, radicle) is adjusted to local conditions inside the seed (Borisjuk et al, Plant Cell 2013, Lorenz et al., J Proteomics 2014, Schwender et al., Plant Physiology 2015). In current research, we focus on the characterization of the transient endosperm compartment, and investigate how it contributes to growth control of embryo.
Metabolic control and sugar-signalling
Seeds determine the reproductive capacity of plants and are vital to their existence. To ensure seed survival and germination, the embryo enters the maturation phase in late development, which encompasses the accumulation of reserve compounds and the acquisition of desiccation tolerance. Trehalose 6‐phosphate (T6P), which functions as a signal for sugar availability in plants, is believed to regulate storage processes in seeds, since disruption of T6P synthesis in Arabidopsis thaliana causes embryo abortion at the onset of seed filling phase. To investigate the role of T6P during seed development, we modulated the T6P content in pea embryos by ectopic expression of T6P synthase (OtsA) or T6P phosphatase (OtsB) genes from E. coli. We already showed that T6P promotes cotyledon growth and starch accumulation in maturing seeds, and that this requires transcriptional induction of auxin biosynthesis. Our data indicate that T6P integrates auxin signalling with sugar availability to facilitate seed filling (Dissertation T. Meitzel 2018; McAdam et al., New Phytologist 2017). Yet, many aspects of this process are still not understood. To fill these gaps, we plan to characterize the phenotypic and metabolic changes in our transgenic plant models via NMR imaging, metabolite profiling and metabolic flux analysis. Furthermore, special emphasis will be put on the role of T6P during seed germination and lipid reserve accumulation in the oil crop Brassica napus.
Role of programmed cell death for seed filling
We elucidated that programmed cell death (PCD) in maternal seed parts is required for endosperm development and seed filling, thus contributing to the control of seed size in cereal grains. The distributions of TUNEL-positive nuclei, expression of PCD-related genes and cascades of caspase-like activities have revealed that each seed tissue follows an individual PCD pattern. Earlier we found that Triticeae-specific Jekyll gene, exclusively expressed in the nucellar projection, is involved in terminal differentiation of the nucellar tissues switching their cell fate to death (Radchuk et al., Plant Cell 2006). Further, we have established that tissue-specific genes encoding vacuolar processing enzyme (VPE) are required for PCD in distinct grain tissues. Using transcriptional and metabolic profiling, flow cytometry, 13C-feeding experiments, histology and nuclear magnetic resonance imaging of grains we demonstrated that PCD in pericarp is required to provide space for the expanding endosperm and embryo (Radchuk et al., New Phytology 2018). PCD in the nucellar projection contributes to nutrient flow towards endosperm and is controlled by expanded VPE2 subfamily in barley (Mascher et al., Nature 2017) and other Triticeae, compared to other Poaceae species. In current research, we elucidate the functional role of specific gene family members of VPE.
In vivo monitoring of development
Our studies promote NMR‐imaging as a versatile analytic tool for developmental biology, potent for in vivo study of the inner life of plants (Borisjuk Habilitation Thesis “The inner life of seed: from seeing to understanding”, Leibniz Universität Hannover/Germany 2017).
A major thrust of developmental biology is to understand how molecular and cellular processes produce 3D morphology. Nuclear Magnetic Resonance Imaging (MRI) has a great virtue in being non‐invasive and therefore has the potential to monitor physiological processes in vivo. In our hands, MRI is capable to capture the previously hidden growth/storage without seed destruction and thus allows us to monitor living seed (Borisjuk et al., Plant J. 2012). We prime MRI for non-invasive visualization and survey of flower/seed interior and apply high resolution chemical (FT IR) imaging to characterize structure and composition of tissues with close to cellular resolution (Gündel et al., Plant Physiology 2018).
The introduction of functional imaging on living seed enables us to display seed growth and to uncover intimate events of the awakening of life during germination (Munz et al., New Phytologist 2017). A holistic in vivo approach was designed to display the link between the entry and allocation of water, metabolic events and structural changes occurring during germination. We uncovered an endospermal lipid gap, which channels water to the radicle tip, from whence it is distributed via embryonic vasculature toward cotyledon tissues. The resumption of respiration, sugar metabolism and lipid utilization are linked to the spatiotemporal sequence of tissue rehydration.
Mechanosensing
It is virtually unknown whether and how morphological changes in the embryo during development influence embryonic metabolism. For example, the curvature of the embryonic axis or the developing cotyledons could change the local growth conditions. It is unclear how the process of embryonic shape formation is perceived and translated into corresponding metabolic signals. The processes involved - termed mechanosensing and proprioception - are being investigated in current DFG projects using the oilseed rape model. In particular, we are looking for mechanisms of how the growing embryo adapts its shape and size to the available space (limited by the seed coat) and how this is reflected in its metabolism.
Other models in which we describe developmental processes are barley, maize, pea, and Arabidopsis (Kovalchuk et al., New Phytologist 2016; Radchuk et al., 2018; Lappe et al., PNAS 2018). In addition, the above-mentioned research approaches and methods are used as part of collaborations with breeders (rapeseed, wheat).
Advancement of the bioanalytical infrastructure
The development of novel bioanalytical methods is an essential part of our work in the various projects funded by DFG and BMBF. In particular, we work on: (1) improvement of imaging tools for plants using NMR/MRI, (2) biochemical methods for micro sampling using novel Orbitrap technology (LC/MS), (3) FTIR-microscopy for the analysis of spatial gradients in sugars, hormones and more, (4) methods for the high throughput screening of seeds using NIRS, TD-NMR and robotized sample delivery systems.
Examples of important method developments are given by Melkus et al., (Plant Biotech J 2011), Fuchs et al. (Plant Physiology 2013), Borisjuk et al. (Plant Cell 2013), Rolletschek et al. (Plant Biotech J 2015), Munz et al. (New Phytologist 2017), and Gündel et al. (Plant Physiology 2018).
scroll top
Staff
scroll top
Publications
Borisjuk L, Neuberger T:
Perspectives: The look insight - magnetic resonance imaging (MRI) of the inner life of plants. J. Plant Physiol. 309 (2025) 154502. https://dx.doi.org/10.1016/j.jplph.2025.154502
Knoch D, Rugen N, Thiel J, Heuermann M C, Kuhlmann M, Rizzo P, Meyer R C, Wagner S, Schippers J H M, Braun H-P, Altmann T:
A spatio-temporal transcriptomic and proteomic dataset of developing Brassica napus seeds. Sci. Data 12 (2025) 759. https://dx.doi.org/10.1038/s41597-025-05115-4
Plutenko I, Radchuk V, Mayer S, Keil P, Ortleb S, Wagner S, Lehmann V, Rolletschek H, Borisjuk L:
MRI-Seed-Wizard: combining deep learning algorithms with magnetic resonance imaging enables advanced seed phenotyping. J. Exp. Bot. 76 (2025) 393-410. https://dx.doi.org/10.1093/jxb/erae408
Rolletschek H, Borisjuk L, Gómez-Álvrez E M, Pucciariello C:
Advances in seed hypoxia research - an updated review. Plant Physiol. 197 (2025) kiae556. https://dx.doi.org/10.1093/plphys/kiae556
Schüler D, Lange M, Altmann T, Cuacos M, Arend D, D’Auria J C, Fiebig A, Kumlehn J, Neumann K, Melzer M, Rey-Mazón E, Rolletschek H, Scholz U, Willner E, Reif J C:
Data management in balance – a decade of balancing pragmatism, sustainability and innovation at plant research center IPK Gatersleben. J. Integr. Bioinform. (2025) Epub ahead of print. https://dx.doi.org/10.1515/jib-2025-0012
Hinrichs P:
Comparative study of maize kernels with magnetic resonance imaging and semantic segmentation. (Master Thesis) Hannover, Gottfried Wilhelm Leibniz Universität Hannover, Naturwissenschaftliche Fakultät (2024) 84 pp.
Langer M:
Investigations on maize kernel development and the relevance of endogenous hypoxia. (PhD Thesis) Hannover, Gottfried Wilhelm Leibniz Universität Hannover, Naturwissenschaftliche Fakultät (2024) 124 pp.
Mayer S, Rolletschek H, Radchuk V, Wagner S, Ortleb S, Gündel A, Dehmer K J, Gutjahr F T, Jakob P M, Borisjuk L:
Metabolic imaging in living plants: A promising field for chemical exchange saturation transfer (CEST) MRI. Sci. Adv. 10 (2024) eadq4424. https://dx.doi.org/10.1126/sciadv.adq4424
Meitzel T:
Good things come to those who wait - a 42-yr study challenges trade-off theories. New Phytol. 241 (2024) 521-522. https://dx.doi.org/10.1111/nph.19350
Rezaeva B R, Rutten T, Bollmann C, Ortleb S, Melzer M, Kumlehn J:
Plant regeneration via adventitious shoot formation from immature zygotic embryo explants of Camelina. Plants 13 (2024) 465. https://dx.doi.org/10.3390/plants13040465
Rolletschek H, Muszynska A, Schwender J, Radchuk V, Heinemann B, Hilo A, Plutenko I, Keil P, Ortleb S, Wagner S, Kalms L, Gündel A, Shi H, Fuchs J, Szymanski J J, Braun H-P, Borisjuk L:
Mechanical forces orchestrate the metabolism of the developing oilseed rape embryo. New Phytol. 244 (2024) 1328-1344. https://dx.doi.org/10.1111/nph.19990
Rutten T, Thirulogachandar V, Huang Y, Shanmugaraj N, Koppolu R, Ortleb S, Hensel G, Kumlehn J, Melzer M, Schnurbusch T:
Anatomical insights into the vascular lay-out of the barley rachis: implications for transport and spikelet connection. Ann. Bot. 133 (2024) 983-996. https://dx.doi.org/10.1093/aob/mcae025
Vogelsang N:
Complementation of the yeast Δtps2 deletion mutant by expression of TPPs involved in wheat floret abortion. (Master Thesis) Köthen, Hochschule Anhalt, Fachbereich Angewandte Biowissenschaften und Prozesstechnik (2024)
Wamhoff D, Gündel A, Wagner S, Ortleb S, Borisjuk L, Winkelmann T:
Anatomical limitations in adventitious root formation revealed by magnetic resonance imaging, infrared spectroscopy, and histology of rose genotypes with contrasting rooting phenotypes. J. Exp. Bot. 75 (2024) 4784-4801. https://dx.doi.org/10.1093/jxb/erae158
Yu P, Li C, Li M, He X, Wang D, Li H, Marcon C, Li Y, Perez-Limón S, Chen X, Delgado-Baquerizo M, Koller R, Metzner R, van Dusschoten D, Pflugfelder D, Borisjuk L, Plutenko I, Mahon A, Resende M F R J, Salvi S, Akale A, Abdalla M, Ahmed M A, Bauer F M, Schnepf A, Lobet G, Heymans A, Suresh K, Schreiber L, McLaughlin C M, Li C, Mayer M, Schön C-C, Bernau V, von Wirén N, Sawers R J H, Wang T, Hochholdinger F:
Seedling root system adaptation to water availability during maize domestication and global expansion. Nat. Genet. 56 (2024) 1245-1256. https://dx.doi.org/10.1038/s41588-024-01761-3
Alomari D Z, Schierenbeck M, Alqudah A M, Alqahtani M D, Wagner S, Rolletschek H, Borisjuk L, Röder M S:
Wheat grains as a sustainable source of protein for health. Nutrients 15 (2023) 4398. https://dx.doi.org/10.3390/nu15204398
Bellin L, Melzer M, Hilo A, Amaya D L G, Keller I, Meurer J, Möhlmann T:
Nucleotide limitation results in impaired photosynthesis, reduced growth and seed yield together with massively altered gene expression. Plant Cell Physiol. 64 (2023) 1494-1510. https://dx.doi.org/10.1093/pcp/pcad063
Borisjuk L, Horn P, Chapman K, Jakob P M, Gündel A, Rolletschek H:
Seeing plants as never before. New Phytol. 238 (2023) 1775-1794. https://dx.doi.org/10.1111/nph.18871
Gündel A:
Multimodal imaging unveils the hidden dimensions of plant physiology: from metabolic landscapes to mechanistic insights. (PhD Thesis) Hannover, Gottfried Wilhelm Leibniz Universität Hannover, Naturwissenschaftliche Fakultät (2023) 240 pp.
Keil P, Gündel B, Gündel A, Rolletschek H, Borisjuk L:
Non-invasive single-grain screening of proteins and other features by combination of near-infrared spectroscopy and nuclear magnetic resonance. Agronomy 13 (2023) 1393. https://dx.doi.org/10.3390/agronomy13051393
Langer M, Hilo A, Guan J-C, Koch K E, Xiao H, Verboven P, Gündel A, Wagner S, Ortleb S, Radchuk V, Mayer S, Nicolai B, Borisjuk L, Rolletschek H:
Causes and consequences of endogenous hypoxia on growth and metabolism of developing maize kernels. Plant Physiol. 192 (2023) 1268-1288. https://dx.doi.org/10.1093/plphys/kiad038
Mira M M, Hill R D, Hilo A, Langer M, Robertson S, Igamberdiev A U, Wilkins O, Rolletschek H, Stasolla C:
Plant stem cells under low oxygen: metabolic rewiring by phytoglobin underlies stem cell functionality. Plant Physiol. 193 (2023) 1416-1432. https://dx.doi.org/10.1093/plphys/kiad344
Plutenko I, Papkov M, Palo K, Parts L, Fishman D:
Metadata improves segmentation through multitasking elicitation. In: Koch L, Cardoso M J, Ferrante E, Kamnitsas K, Islam M, Jiang M, Rieke N, Tsaftaris S A, Yang D (Eds.): Domain adaptation and representation transfer. DART 2023. (Series: Lecture Notes in Computer Science, Vol. 14293) Cham: Springer (2023) ISBN 978-3-319-23107-5, 147-155. https://dx.doi.org/10.1007/978-3-031-45857-6_15
Radchuk V, Belew Z M, Gündel A, Mayer S, Hilo A, Hensel G, Sharma R, Neumann K, Ortleb S, Wagner S, Muszynska A, Crocoll C, Xu D, Hoffie I, Kumlehn J, Fuchs J, Peleke F F, Szymanski J J, Rolletschek H, Nour-Eldin H H, Borisjuk L:
SWEET11b transports both sugar and cytokinin in developing barley grains. Plant Cell 35 (2023) 2186-2207. https://dx.doi.org/10.1093/plcell/koad055
Shi H, Ernst E, Heinzel N, McCorkle S, Rolletschek H, Borisjuk L, Ortleb S, Martienssen R, Shanklin J, Schwender J:
Mechanisms of metabolic adaptation in the duckweed Lemna gibba: an integrated metabolic, transcriptomic and flux analysis. BMC Plant Biol. 23 (2023) 458. https://dx.doi.org/10.1186/s12870-023-04480-9
Teh J T, Leitz V, Holzer V J C, Neusius D, Marino G, Meitzel T, García-Cerdán J G, Dent R M, Niyogi K K, Geigenberger P, Nickelsen J:
NTRC regulates CP12 to activate Calvin-Benson cycle during cold acclimation. Proc. Natl. Acad. Sci. U.S.A. 120 (2023) e2306338120. https://dx.doi.org/10.1073/pnas.2306338120
Blume R Y, Yemets A I, Korkhovyi V, Radchuk V, Rakhmetov D, Blume Y B:
Genome-wide identification and analysis of cytokinin oxidase/dehydrogenase (ckx) gene family in finger millet (Eleusine coracana) Front. Genet. 13 (2022) 963789. https://dx.doi.org/10.3389/fgene.2022.963789
Bouquet F:
Mechanismen der molekularen und biochemischen Anpassung zu Hypoxie in Maissamen. (Master Thesis) Hannover, Gottfried Wilhelm Leibniz Universität Hannover, Naturwissenschaftliche Fakultät, Institut für Pflanzengenetik (2022) 86 pp.
Mayer S, Munz E, Hammer S, Wagner S, Guendel A, Rolletschek H, Jakob P M, Borisjuk L, Neuberger T:
Quantitative monitoring of paramagnetic contrast agents and their allocation in plant tissues via DCE-MRI. Plant Methods 18 (2022) 47. https://dx.doi.org/10.1186/s13007-022-00877-z
Nagel M, Arc E, Rajjou L, Cueff G, Bailly M, Clément G, Sanchez-Vicente I, Bailly C, Seal C E, Roach T, Rolletschek H, Lorenzo O, Börner A, Kranner I:
Impacts of drought and elevated temperature on the seeds of malting barley. Front. Plant Sci. 13 (2022) 1049323. https://dx.doi.org/10.3389/fpls.2022.1049323
Plaehn N M J, Mayer S, Jakob P M, Gutjahr F T:
T1-independent exchange rate quantification using saturation- or phase sensitive-water exchange spectroscopy. J. Magn. Reson. 335 (2022) 107141. https://dx.doi.org/10.1016/j.jmr.2021.107141
Schüler V J:
Analyse des Interaktionsmechanismus des Nuclear Factor Y Komplexes in Gerstenkörnern. (Bachelor Thesis) Köthen, Hochschule Anhalt, Fachbereich Angewandte Biowissenschaften und Prozesstechnik (2022) 61 pp.
Acosta K, Appenroth K J, Borisjuk L, Edelman M, Heinig U, Jansen M A K, Oyama T, Pasaribu B, Schubert I, Sorrels S, Sree K S, Xu S, Michael T P, Lam E:
Return of the Lemnaceae: Duckweed as a model plant system in the genomics and post-genomics era. Plant Cell 33 (2021) 3207-3234. https://dx.doi.org/10.1093/plcell/koab189
Fiebelkow J, Guendel A, Guendel B, Mehwald N, Jetka T, Komorowski M, Waldherr S, Schaper F, Dittrich A:
The tyrosine phosphatase SHP2 increases robustness and information transfer within IL-6-induced JAK/STAT signalling. Cell Commun. Signal. 19 (2021) 94. https://dx.doi.org/10.1186/s12964-021-00770-7
Goelckel L:
Cellular plasticity and mechanosensing in the Brassica napus embryo. (Master Thesis) Halle/S., Martin-Luther-Universität Halle-Wittenberg, Naturwissenschaftliche Fakultät I Biowissenschaften, Institut für Biologie (2021) 61 pp.
Gomez-Sanchez A, Santamaria M E, Gonzalez-Melendi P, Muszynska A, Matthess C, Martinez M, Diaz I:
Repression of barley cathepsins, HvPap-19 and HvPap-1, differentially alters grain composition and delays germination. J. Exp. Bot. 72 (2021) 3474-3485. https://dx.doi.org/10.1093/jxb/erab007
Guendel A, Hilo A, Rolletschek H, Borisjuk L:
Probing the metabolic landscape of plant vascular bundles by infrared fingerprint analysis, imaging and mass spectrometry. Biomolecules 11 (2021) 1717. https://dx.doi.org/10.3390/biom11111717
Meitzel T, Radchuk R, McAdam E L, Thormählen I, Feil R, Munz E, Hilo A, Geigenberger P, Ross J J, Lunn J E, Borisjuk L:
Trehalose 6-phosphate promotes seed filling by activating auxin biosynthesis. New Phytol. 229 (2021) 1553-1565. https://dx.doi.org/10.1111/nph.16956
Michael T P, Ernst E, Hartwick N, Chu P, Bryant D, Gilbert S, Ortleb S, Baggs E L, Sree K S, Appenroth K J, Fuchs J, Jupe F, Sandoval J P, Krasileva K V, Borisjuk L, Mockler T C, Ecker J, Martienssen R A, Lam E:
Genome and time-of-day transcriptome of Wolffia australiana link morphological minimization with gene loss and less growth control. Genome Res. 31 (2021) 225-238. https://dx.doi.org/10.1101/gr.266429.120
Muszynska A, Guendel A, Melzer M, Tandrón Moya Y, Röder M, Rolletschek H, Rutten T, Munz E, Melz G, Ortleb S, Borisjuk L, Börner A:
A mechanistic view on lodging resistance in rye and wheat: a multiscale comparative study. Plant Biotechnol. J. 19 (2021) 2646-2661. https://dx.doi.org/10.1111/pbi.13689
Radchuk V, Tran V, Hilo A, Muszynska A, Gündel A, Wagner S, Fuchs J, Hensel G, Ortleb S, Munz E, Rolletschek H, Borisjuk L:
Grain filling in barley relies on developmentally controlled programmed cell death. Commun. Biol. 4 (2021) 428. https://dx.doi.org/10.1038/s42003-021-01953-1
Rolletschek H:
Hardy Rolletschek. New Phytol. 232 (2021) 476-478. https://dx.doi.org/10.1111/nph.17567
Rolletschek H, Mayer S, Boughton B, Wagner S, Ortleb S, Kiel C, Roessner U, Borisjuk L:
The metabolic environment of the developing embryo: A multidisciplinary approach on oilseed rapeseed. J. Plant Physiol. 265 (2021) 153505. https://dx.doi.org/10.1016/j.jplph.2021.153505
Rolletschek H, Muszynska A, Borisjuk L:
The process of seed maturation is influenced by mechanical constraints. New Phytol. 229 (2021) 19-23. https://dx.doi.org/10.1111/nph.16815
Vogelsang N:
A novel sensor to map the trehalose 6-phosphate distribution in planta. (Bachelor Thesis) Köthen, Hochschule Anhal (2021) 43 pp.
Borisjuk L, Rolletschek H, Radchuk V:
Advances in understanding of barley plant physiology: factors determining grain development and composition/chemistry. In: Fox G, Li C (Eds.): Achieving sustainable cultivation of barley. (Burleigh Dodds Series in Agricultural Science, Vol. 74) Cambridge, UK: Burleigh Dodds (2020) ISBN 978-1-78676-308-2, 53-96. https://dx.doi.org/10.19103/AS.2019.0060.03
Impe D, Reitz J, Köpnick C, Rolletschek H, Börner A, Senula A, Nagel M:
Assessment of pollen viability for wheat. Front. Plant Sci. 10 (2020) 1588. https://dx.doi.org/10.3389/fpls.2019.01588
Isayenkov S, Hilo A, Rizzo P, Tandron Moya Y A, Rolletschek H, Borisjuk L, Radchuk V:
Adaptation strategies of halophytic barley Hordeum marinum spp marinum to high salinity and osmotic stress. Int. J. Mol. Sci. 21 (2020) 9019. https://dx.doi.org/10.3390/ijms21239019
Le H, Nguyen N H, Ta D T, Le T N T, Bui T P, Le N T, Nguyen C X, Rolletschek H, Stacey G, Stacey M G, Pham N B, Do P T, Chu H H:
CRISPR/Cas9-mediated knockout of galactinol synthase-encoding genes reduces raffinose family oligosaccharide levels in soybean seeds. Front. Plant Sci. 11 (2020) 612942. https://dx.doi.org/10.3389/fpls.2020.612942
Rolletschek H, Schwender J, König C, Chapman K D, Romsdahl T, Lorenz C, Braun H P, Denolf P, van Audenhove K, Munz E, Heinzel N, Ortleb S, Rutten T, McCorkle S, Borysyuk T, Gündel A, Shi H, Vander Auwermeulen M, Bourot S, Borisjuk L:
Cellular plasticity in response to suppression of storage proteins in the Brassica napus embryo. Plant Cell 32 (2020) 2383-2401. https://dx.doi.org/10.1105/tpc.19.00879
Stickel F:
Phänotypische, biochemische und molekulare Untersuchungen von Hordeum vulgare mit modulierter Expression von SWEET-Transportern. (Bachelor Thesis) Hannover, Gottfried Wilhelm Leibniz Universität Hannover, Naturwissenschaftliche Fakultät (2020) 90 pp.
Sturtevant D, Lu S, Zhou Z W, Shen Y, Wang S, Song J M, Zhong J, Burks D J, Yang Z Q, Yang Q Y, Cannon A E, Herrfurth C, Feussner I, Borisjuk L, Munz E, Verbeck G F, Wang X, Azad R K, Singleton B, Dyer J M, Chen L L, Chapman K D, Guo L:
The genome of jojoba (Simmondsia chinensis): A taxonomically isolated species that directs wax ester accumulation in its seeds. Sci. Adv. 6 (2020) eaay3240. https://dx.doi.org/10.1126/sciadv.aay3240
Tikhenko N, Alqudah A M, Borisjuk L, Ortleb S, Rutten T, Wu D D, Nagel M, Himmelbach A, Mascher M, Röder M, Ganal M, Sehmisch S, Houben A, Börner A:
DEFECTIVE ENDOSPERM-D1 (Dee-D1) is crucial for endosperm development in hexaploid wheat. Commun. Biol. 3 (2020) 791. https://doi.org/10.1038/s42003-020-01509-9
Druege U, Hilo A, Pérez-Pérez J M, Klopotek Y, Acosta M, Shahinnia F, Zerche S, Franken P, Hajirezaei M R:
Molecular and physiological control of adventitious rooting in cuttings: phytohormone action meets resource allocation. Ann. Bot. 123 (2019) 929–949. https://dx.doi.org/10.1093/aob/mcy234
Gündel A, Rolletschek H, Borisjuk L:
Infrared visualization of sucrose in plants. A novel microspectroscopic method allows sugar mapping across the plant tissue. q-more.chemeurope.com/q-more-articles/279/infrared-visualization-of-sucrose-in-plants.html. (2019)
Gündel A, Rolletschek H, Wagner S, Muszynska A, Borisjuk L:
More insights with infrared. New method enables quantitative visualization of sucrose distribution in plants. Imaging & Microscopy 1 (2019) 28-29.
Gündel A, Rolletschek H, Wagner S, Muszynska A, Borisjuk L:
Mehr sehen mit Infrarot – Quantitative Visualisierung der Saccharose-Verteilung in Pflanzen. GIT Labor-Fachzeitschr. 63 (2019) 38-39.
Gutjahr F T, Munz E, Jakob P M:
Positive chemical exchange contrast in MRI using Refocused Acquisition of Chemical Exchange Transferred Excitations (RACETE). Z. Med. Phys. 29 (2019) 184-191. https://dx.doi.org/10.1016/j.zemedi.2018.05.005
Langer M:
Untersuchungen zur Samenentwicklung bei Cyclamen persicum MILL. – Visualisierung über NMR sowie Lipid- und Phytohormonanalysen. (Master Thesis) Hannover, Gottfried Wilhelm Leibniz Universität Hannover, Naturwissenschaftliche Fakultät (2019) 126 pp.
Radchuk V, Sharma R, Potokina E, Radchuk R, Weier D, Munz E, Schreiber M, Mascher M, Stein N, Wicker T, Kilian B, Borisjuk L:
The highly divergent Jekyll genes, required for sexual reproduction, are lineage specific for the related grass tribes Triticeae and Bromeae. Plant J. 98 (2019) 961-974. https://dx.doi.org/10.1111/tpj.14363
Rizzo P, Altschmied L, Stark P, Rutten T, Guendel A, Scharfenberg S, Franke K, Baeumlein H, Wessjohann L, Koch M, Borisjuk L, Sharbel T F:
Discovery of key regulators of dark glands development and hypericin biosynthesis in St. Johns wort (Hypericum perforatum). Plant Biotechnol. J. 17 (2019) 2299-2312. https://dx.doi.org/10.1111/pbi.13141
Savchenko T, Rolletschek H, Dehesh K:
Jasmonates-mediated rewiring of central metabolism regulates adaptive responses. Plant Cell Physiol. 60 (2019) 2613-2620. https://dx.doi.org/10.1093/pcp/pcz181
Savchenko T, Rolletschek H, Heinzel N, Tikhonov K, Dehesh K:
Waterlogging tolerance rendered by oxylipin-mediated metabolic reprogramming in Arabidopsis. J. Exp. Bot. 70 (2019) 2919–2932. https://dx.doi.org/10.1093/jxb/erz110
Tedeschi F, Rizzo P, Huong B, Czihal A, Rutten T, Altschmied L, Scharfenberg S, Grosse I, Becker C, Weigel D, Bäumlein H, Kuhlmann M:
EFFECTOR OF TRANSCRIPTION factors are novel plant-specific regulators associated with genomic DNA methylation in Arabidopsis. New Phytol. 221 (2019) 261-278. https://dx.doi.org/10.1111/nph.15439
Wabila C, Neumann K, Kilian B, Radchuk V, Graner A:
A tiered approach to genome-wide association analysis for the adherence of hulls to the caryopsis of barley seeds reveals footprints of selection. BMC Plant Biol. 19 (2019) 95. https://dx.doi.org/10.1186/s12870-019-1694-1
Zornow R:
Untersuchungen zum Keimverhalten von Getreide- und Ölsaaten. (Master Thesis) Hannover, Gottfried Wilhelm Leibniz Universität Hannover, Naturwissenschaftliche Fakultät (2019) 99 pp.
Gündel A, Rolletschek H, Wagner S, Muszynska A, Borisjuk L:
Micro imaging displays the sucrose landscape within and along its allocation pathways. Plant Physiol. 178 (2018) 1448-1460. https://dx.doi.org/10.1104/pp.18.00947
Lappe R R, Baier J W, Boehlein S K, Huffman R, Lin Q, Wattebled F, Settles A M, Hannah L C, Borisjuk L, Rolletschek H, Stewart J D, Scott M P, Hennen-Bierwagen T A, Myers A M:
Functions of maize genes encoding pyruvate phosphate dikinase in developing endosperm. Proc. Natl. Acad. Sci. U.S.A. 115 (2018) E24-E33. https://dx.doi.org/10.1073/pnas.1715668115
Lorenz C, Brandt S, Borisjuk L, Rolletschek H, Heinzel N, Tohge T, Fernie A R, Braun H-P, Hildebrandt T M:
The role of persulfide metabolism during Arabidopsis seed development under light and dark conditions. Front. Plant Sci. 9 (2018) 1381. https://dx.doi.org/10.3389/fpls.2018.01381
Meitzel T:
Signaling pathways in legume seed development: evidence for a crosstalk between trehalose 6-phosphate and auxin. (PhD Thesis) Halle/S., Martin-Luther-Universität Halle-Wittenberg, Naturwissenschaftliche Fakultät I Biowissenschaften (2018) 177 pp.
Munz E:
High resolution, physiological and metabolic MRI of plants. (PhD Thesis) Würzburg, Julius-Maximilians-Universität (2018) 170 pp.
Muszynska A:
Histological, ultrastructural, elemental, and molecular genetic characterization of ′Stabilstroh’, a complex trait of rye (Secale cereale L.) determining lodging resistance. (PhD Thesis) Halle/S., Martin-Luther-Universität Halle-Wittenberg, Naturwissenschaftliche Fakultät III Agrar- und Ernährungswissenschaften, Geowissenschaften und Informatik (2018) 161 pp.
Radchuk V, Tran V, Radchuk R, Diaz-Mendoza M, Weier D, Fuchs J, Riewe D, Hensel G, Kumlehn J, Munz E, Heinzel N, Rolletschek H, Martinez M, Borisjuk L:
Vacuolar processing enzyme 4 contributes to maternal control of grain size in barley by executing programmed cell death in the pericarp. New Phytol. 218 (2018) 1127-1142. https://dx.doi.org/10.1111/nph.14729
Rajaraman J, Douchkov D, Lück S, Hensel G, Nowara D, Pogoda M, Rutten T, Meitzel T, Brassac J, Höfle C, Hückelhoven R, Klinkenberg J, Trujillo M, Bauer E, Schmutzer T, Himmelbach A, Mascher M, Lazzari B, Stein N, Kumlehn J, Schweizer P:
Evolutionarily conserved partial gene duplication in the Triticeae tribe of grasses confers pathogen resistance. Genome Biol. 19 (2018) 116. https://dx.doi.org/10.1186/s13059-018-1472-7
Walerowski P, Gündel A, Yahaya N, Truman W, Sobczak M, Olszak M, Rolfe S A, Borisjuk L, Malinowski R:
Clubroot disease stimulates early steps of phloem differentiation and recruits SWEET sucrose transporters within developing galls. Plant Cell 30 (2018) 3058-3073. https://dx.doi.org/10.1105/tpc.18.00283
Borisjuk L:
The inner life of seed: from seeing to understanding. (Habilitation Thesis) Hannover, Gottfried Wilhelm Leibniz Universität Hannover, Naturwissenschaftliche Fakultät (2017) 402 pp.
Keil P, Liebsch G, Borisjuk L, Rolletschek H:
MultiSense: a multimodal sensor tool enabling the high throughput analysis of respiration. In: Kapuganti J G (Ed.): Plant respiration and internal oxygen: methods and protocols. (Series: Methods in molecular biology, Vol. 1670) New York, NY [u.a.]: Humana Press (2017) ISBN 978-1-4939-7291-3, 47-56. https://dx.doi.org/10.1007/978-1-4939-7292-0_5
König C:
Molecular and metabolic characterization of assimilate uptake and storage product synthesis in Brassica napus. (PhD Thesis) Hannover, Gottfried Wilhelm Leibniz Universität Hannover, Naturwissenschaftliche Fakultät (2017) 119 pp.
Mascher M, Gundlach H, Himmelbach A, Beier S, Twardziok S O, Wicker T, Radchuk V, Dockter C, Hedley P E, Russell J, Bayer M, Ramsay L, Liu H, Haberer G, Zhang X-Q, Zhang Q, Barrero R A, Li L, Taudien S, Groth M, Felder M, Hastie A, Šimková H, Staňková H, Vrána J, Chan S, Muñoz-Amatriaín M, Ounit R, Wanamaker S, Bolser D, Colmsee C, Schmutzer T, Aliyeva-Schnorr L, Grasso S, Tanskanen J, Chailyan A, Sampath D, Heavens D, Clissold L, Cao S, Chapman B, Dai F, Han Y, Li H, Li X, Lin C, McCooke J K, Tan C, Wang P, Wang S, Yin S, Zhou G, Poland J A, Bellgard M I, Borisjuk L, Houben A, Doležel J, Ayling S, Lonardi S, Kersey P, Langridge P, Muehlbauer G J, Clark M D, Caccamo M, Schulman A H, Mayer K F X, Platzer M, Close T J, Scholz U, Hansson M, Zhang G, Braumann I, Spannagl M, Li C, Waugh R, Stein N:
A chromosome conformation capture ordered sequence of the barley genome. Nature 544 (2017) 427-433. https://dx.doi.org/10.1038/nature22043
McAdam E L, Meitzel T, Quittenden L J, Davidson S E, Dalmais M, Bendahmane A I, Thompson R, Smith J J, Nichols D S, Urquhart S, Gélinas-Marion A, Aubert G, Ross J J:
Evidence that auxin is required for normal seed size and starch synthesis in pea. New Phytol. 216 (2017) 193-204. https://dx.doi.org/10.1111/nph.14690
Munz E, Rolletschek H, Oeltze-Jafra S, Fuchs J, Guendel A, Neuberger T, Ortleb S, Jakob P M, Borisjuk L:
A functional imaging study of germinating oilseed rape seed. New Phytol. 216 (2017) 1181-1190. https://dx.doi.org/10.1111/nph.14736
Radchuk V, Riewe D, Peukert M, Matros A, Strickert M, Radchuk R, Weier D, Steinbiß H-H, Sreenivasulu N, Weschke W, Weber H:
Down-regulated sucrose transporters HvSUT1, HvSUT2 affects sucrose homeostasis along its delivery path in barley grains. J. Exp. Bot. 68 (2017) 4595-4612. https://doi.org/10.1093/jxb/erx266
Reichelt W N, Brillmann M, Thurrold P, Keil P, Fricke J, Herwig C:
Physiological capacities decline during induced bioprocesses leading to substrate accumulation. In: Ferreira G N M, Glassey J (Eds.): Biotechnol. J. (Special Issue: European Symposium on Biochemical Engineering Science, Dublin 2016). (Vol. 7) : WILEY-VCH Verlag (2017) 1860-7314, 1600547. https://dx.doi.org/10.1002/biot.201600547
Rolletschek H, Borisjuk L, Hennen-Bierwagen T A, Myers A M:
Central metabolism and its spatial heterogeneity in maize endosperm. In: Larkins B A (Ed.): Maize kernel development. Boston, MA: CABI (2017) ISBN 978-1-78639-121-6, 134-148.
Rolletschek H, Liebsch G:
A method for imaging oxygen distribution and respiration at a microscopic level of resolution. In: Kapuganti J G (Ed.): Plant respiration and internal oxygen: methods and protocols. (Series: Methods in molecular biology, Vol. 1670) New York, NY [u.a.]: Humana Press (2017) ISBN 978-1-4939-7291-3, 31-38. https://dx.doi.org/10.1007/978-1-4939-7292-0_3
Scherzer S, Shabala L, Hedrich B, Fromm J, Bauer H, Munz E, Jakob P, Al-Rascheid K A S, Kreuzer I, Becker D, Eiblmeier M, Rennenberg H, Shabala S, Bennett M, Neher E, Hedrich R:
Insect haptoelectrical stimulation of Venus flytrap triggers exocytosis in gland cells. Proc. Natl. Acad. Sci. U.S.A. 114 (2017) 4822-4827. https://dx.doi.org/10.1073/pnas.1701860114
Woodfield H K, Sturtevant D, Borisjuk L, Munz E, Guschina I A, Chapman K D, Harwood J L:
Spatial and temporal mapping of key lipid species in Brassica napus seeds. Plant Physiol. 173 (2017) 1998-2009. https://dx.doi.org/10.1104/pp.16.01705
Keller E R J, Grübe M, Hajirezaei M-R, Melzer M, Mock H-P, Rolletschek H, Senula A, Subbarayan K:
Experience in large-scale cryopreservation and links to applied research for safe storage of plant germplasm. In: Lambardi M, Hamill S (Eds.): Proceedings of the XXIX IHC - Int. Symp. on Micropropagation and In Vitro Techniques, Brisbane, Australia, August 17-22, 2014. (Series: Acta Horticulturae, Vol. 1113) Leuven: ISHS (2016) 239-249. https://dx.doi.org/10.17660/ActaHortic.2016.1113.36
Kovalchuk N, Chew W, Sornaraj P, Borisjuk N, Yang N, Singh R, Bazanova N, Shavrukov Y, Guendel A, Munz E, Borisjuk L, Langridge P, Hrmova M, Lopato S:
The homeodomain transcription factor TaHDZipI-2 from wheat regulates frost tolerance, flowering time and spike development in transgenic barley. New Phytol. 211 (2016) 671-687. https://dx.doi.org/10.1111/nph.13919
Munz E, Jakob P M, Borisjuk L:
The potential of nuclear magnetic resonance to track lipids in planta. Biochimie 130 (2016) 97-108. https://dx.doi.org/10.1016/j.biochi.2016.07.014
scroll top
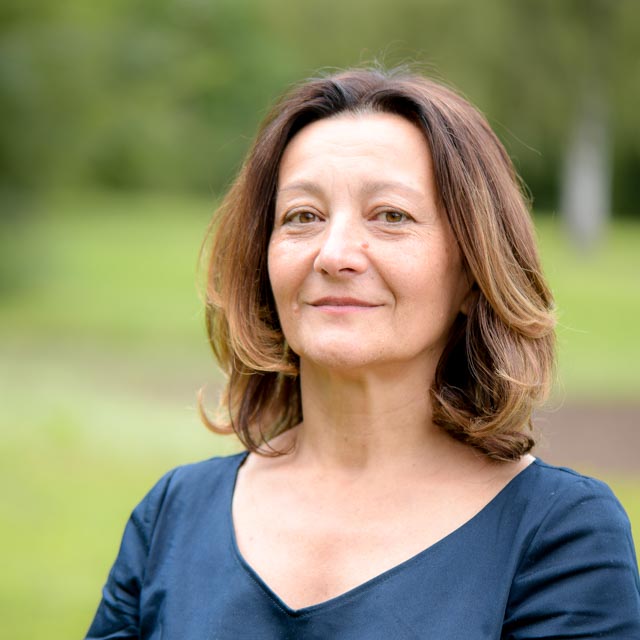